Terrance Cooper, PhD
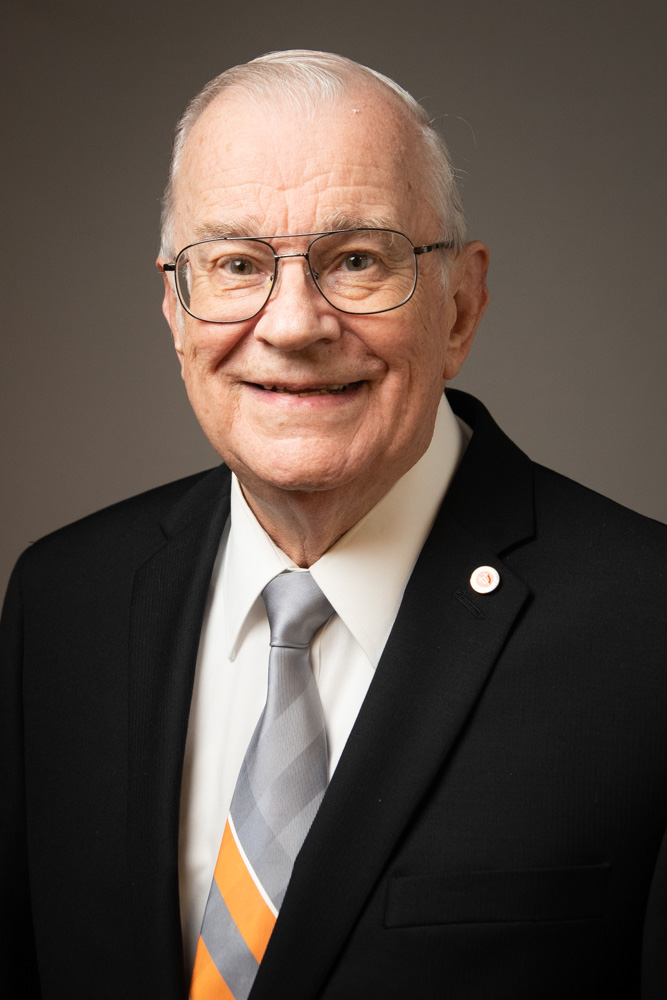
Hariett S. Van Vleet Professor of Microbiology and Immunology
858 Madison Ave.
501 Molecular Science Building
Memphis, TN 38163
tcooper@uthsc.edu
Phone: 901.448.6179
Fax: 901.448.7360
Research Interests
The focus of our laboratory, The UT Yeast Group –The importance and increasing clinical use of rapamycin (Rap-) family drugs to treat tissue rejection, multiple cancers and potentially progeria necessitate that we accurately and completely understand how the target of these drugs, the mammalian Target Of Rapamycin Complex 1 (mTorC1), is regulated and in turn how it regulates downstream cellular processes. For example, an incomplete understanding of how mTorC1 regulates downstream events (increases Tissue Factor production which triggers the coagulation cascade) has raised concern about late stent thrombosis in rapamycin-derivative eluting stents used to treat acute myocardial infarction. Despite impressive advances, one of the least well understood aspects of
TorC1 (yeast) and mTorC1 (mammals) regulation are the mechanisms of its sensing of and responses to nitrogenous compounds. To address this important problem, we are investigating the mechanisms of nitrogen-responsive GATA factor (Gln3 and Gat1) phosphorylation, localization and transcriptional regulation, which have been (since 1999) and continue to be one of the most widely used reporters of TorC1 activity in pathogenic and non-pathogenic yeasts.
The significance of our most recent work is that hypothesis-driven mechanistic experiments clearly demonstrate significant inconsistencies between predictions of the TorC1 regulatory paradigm and observed experimental data. These results clearly demonstrate a need to revise the paradigm and provide the means to do so. We have developed the information, genetic, cell biological and biochemical tools required to generate a more accurate and detailed understanding of nitrogen-responsive GATA factor regulation. Future work will continue to test and flesh out our current working hypothesis that nitrogen-responsive regulation occurs via multiple pathways with distinct molecular inputs, responses and regulatory protein requirements rather than a single input driving multiple outputs via TorC1 activity. If our ideas are correct, they will ultimately prevent molecular events and mechanisms from being erroneously attributed to mTorC1 that are in reality derived from other sources.
Overview of GATA factor regulation –The highly conserved GATA-family DNA-binding proteins contain a characteristic C-4 zinc-finger (C-X2-C-N17-20-C-X2-C) motif that binds to GATA sequences situated in promoters of regulated genes. [The photograph shows a GATA factor fused to GFP and bound to the 16 yeast chromosomes of a dividing yeast cell.] Only Gln3 and Gat1 of the 8 GATA-family proteins in S. cerevisiae are of interest here. A major function of Gln3 and Gat1 is to activate nitrogen-responsive transcription needed to exploit poor nitrogen sources in the environment when nothing better is available. In nitrogen-excess (e.g. glutamine medium), Gln3 is cytoplasmic and transcription of genes encoding permeases and enzymes required to degrade poor nitrogen sources (e.g. proline) is repressed. The negative regulator Ure2 is required for cytoplasmic sequestration of Gln3 under these conditions. In contrast, when good nitrogen sources are limiting or only poor sources are available, Gln3 moves to the nucleus and transcription of these nitrogen-responsive genes dramatically increases.
TorC1 regulation of Gln3 & Gat1 localization – The pivotal correlations that established the paradigm for Tor regulation of Gln3 were that treating YPD-grown wild type cells with Rap induced: (i) Gln3 dephosphorylation, (ii) dissociation of a Ure2-Gln3 complex, nuclear Gln3 localization and (iii) high level Gln3-mediated transcription. In contrast, in similarly grown, untreated cells Gln3 was phosphorylated, complexed with Ure2, localized to the cytoplasm, and GATA factor-mediated transcription was minimal. Gat1 was reported to be regulated in the same way. Methionine sulfoximine (Msx), an inhibitor of glutamine synthetase, similarly induced nuclear Gln3 localization and derepressed transcription leading to the suggestion that glutamine or a related metabolite was responsible for signaling TorC1 that nitrogen was in excess. In the original model Tap42 complexed with and was posited to be a negative regulator of the Tor pathway phosphatase Sit4, which contradicted Arndt’s earlier genetic report that Tap42 was a positive phosphatase regulator. The conflicting reports were rectified by the finding that an inactive Tap42-Sit4 complex is bound to TorC1. Rap-treatment dissociates the Tap42-Sit4 complex from TorC1 thereby activating Sit4. Subsequently, the active Tap42-Sit4 complex dissociates thereby inactivating Sit4. However, both models posit the same downstream events, i.e., treating cells with Rap relieves Sit4 inhibition and active Sit4 dephosphorylates Gln3, triggers its dissociation from Ure2, permitting nuclear entry and nitrogen-responsive transcription.
Summary of our most recent results – Over the past several years we have investigated inconsistencies in the Tor pathway by determining the requirements of Sit4 and PP2A phosphatases for responses of Gln3 and Gat1 phosphorylation, intracellular localization and Gln3/Gat1-mediated transcription to Rap, Msx, and growth in excess (glutamine) vs. limiting nitrogen (proline). Our pivotal data led to the current proposal that Gln3 and Gat1 are controlled by at least 2 different regulatory pathways (Figure): The 1st is TorC1-dependent, highly induced by Rap and insensitive to Msx (limiting glutamine), whereas the 2nd is TorC1-independent, and highly induced by nitrogen-limitation or Msx. Gln3 and Gat1 each respond to both pathway signals, but to very different degrees and with different phosphatase requirements. The figure below shows clear distinctions between Gln3 and Gat1 responses to these pathways, i.e. conditions where they are directed to opposite cellular compartments. Gln3 responds strongly to the 2nd pathway but only modestly to 1st. Further, limiting nitrogen- and Msx-induced nuclear Gln3 localization exhibits no detectable PP2A requirement. Conversely, Gat1 responds strongly to Rap (1st pathway) but only weakly to limiting nitrogen and hardly, if at all, to Msx. Further, nuclear Gat1 localization exhibits an absolute requirement for PP2A in limiting nitrogen with or without Rap. We demonstrated for the first time: (i) Rap-induced Gln3 dephosphorylation alone is insufficient to elicit its nuclear localization, (ii) the PP2A requirements for Gln3 and Gat1 phosphorylation and localization in response to limiting nitrogen, Msx and Rap signals, (iii) an opposing relationship exists for the phosphatase requirements of Rap-induced nuclear Gln3 and Gat1 localization as a function of nitrogen availability, (iv) Msx and Rap inhibit different pathways rather than sequential steps of a single linear pathway, and (v) beyond its known and newly identified cytoplasmic functions, PP2A plays a critical intra-nuclear role, being required for GATA factor binding to promoter DNA. If our ideas are correct, and our initial data strongly support them, significant reconsideration of a substantial amount of existing TorC1 & mTorC1 data will be required and new pathways of nutrient-responsive regulation identified in yeast and mammalian cells.
Selected Publications
Selected Publications (from 203 primary, peer-reviewed publications)
- Georis, Tate, Cooper, Dubois (2011) Nitrogen-responsive regulation of GATA family activators, Gln3 and Gat1 0ccurs by two distinct pathways, one inhibited by rapamycin and the other by methionine sulfoximine.J. Biol. Chem. Epub. 10-28-11.
- Georis, Tate, Feller, Cooper, Dubois (2011) Intranuclear function for protein phosphatase PP2A: Pph21 and Pph22 are required for rapamycin-induced GATA factor binding to the DAL5 promoter in yeast.Mol. Cell. Biol. 31:92-104 PMID: 20974806.
- Tate, Georis, Dubois, Cooper (2010) Distinct phosphatase requirements and GATA factor responses to nitrogen catabolite repression and rapamycin treatment in Saccharomyces cerevisiae. J. Biol. Chem. 285:17880-17895. PMID: 20378536.
- Tate, Georis, Feller, Dubois, Cooper (2009) Rapamycin-induced Gln3 dephosphorylation is insufficient for nuclear localization: Sit4 and PP2A phosphatases are regulated and function differently. J. Biol. Chem. 284:2522-2534. PMID: 19015262.
- Georis Tate Cooper Dubois (2008) Tor pathway control of the nitrogen-responsive DAL5 gene bifurcates at the level of Gln3 and Gat1 regulation in Saccharomyces cerevisiae. J. Biol. Chem. 283:8919-8929. PMID: 18245087.
- Tate, Cooper (2007) Stress-responsive Gln3 localization in Saccharomyces cerevisiae is separable from and can overwhelm nitrogen source regulation. J. Biol. Chem. 282:18467-18480. PMID: 17439949.
- Tate, Feller, Dubois, Cooper (2006) Saccharomyces cerevisiae Sit4 phosphatase is active irrespective of the nitrogen source provided, and Gln3 phosphorylation levels become nitrogen source-responsive in a sit4-deleted strain. J. Biol. Chem. 281:37980-37992. PMID: 17015442.
- Tate, Rai, Cooper (2006) Ammonia-specific regulation of Gln3 localization in Saccharomyces cerevisiae by protein kinase Npr1. J. Biol. Chem. 281:28460-28469. PMID: 16864577.
- Kulkarni, Buford, Rai, Cooper (2006) Differing responses of Gat1 and Gln3 phosphorylation and localization to rapamycin and methionine sulfoximine treatment in Saccharomyces cerevisiae. FEMS Yeast Res. 6:218-229. PMID: 16487345.
- Tate, Rai, Cooper (2005) Methionine sulfoximine-treatment and carbon starvation elicit Snf1-independent phosphorylation of the transcriptional activator Gln3 in Saccharomyces cerevisiae. J. Biol. Chem. 280:27195-27204. PMID: 15911613.